Influence oxidation resistance of graphite--graphitization catalysts conclusion
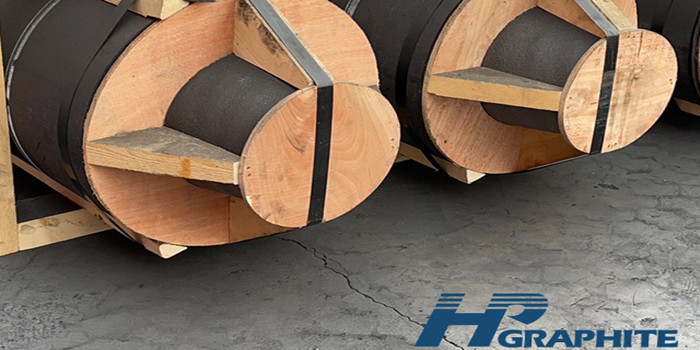
Influence oxidation resistance of graphite--graphitization catalysts conclusion
1. The influence of H3BO3 on the oxidation resistance of graphite
A graphite sample containing B catalyst was prepared using the same process as the above. Among them, H3BO3 was produced by Shanghai Aladdin Biochemical Technology Co., Ltd. AR: ≥99.5% View related graphite electrode products.
As shown in Figure 6(a), as a whole, there is no obvious grain boundary oxidation after oxidation of the graphite sample containing H3BO3 additives, and the surface is still uniform; the white border area in Figure 6(a) is enlarged to obtain Figure 6(b) It can be seen that there is a glassy film scattered on the surface of the crystal grains in dots, and the surface is smooth, and there is no dent formed by oxidation. This shows that B is evenly distributed in graphite, and B2O3 is formed after oxidation, which is adsorbed on graphite crystals as a glassy film. These glassy films cover the active sites of graphite, isolate the graphite matrix from oxygen, and play a role in inhibiting oxidation.
The sample XRD data is shown in Figure 7, and Table 3 lists the lattice parameters of the graphite material. It can be seen that graphite will first decrease and then increase with the increase of H3BO3 addition. When the addition of H3BO3 is 3.0%, d002 reaches the minimum value of 0.3359 nm, and the graphitization degree is the highest of 95.07%. B obviously played a role in catalyzing graphitization.
According to the position of B, B has different effects on the free valence, and B generates free valence at the edge position, causing the formation of C-C bond in the benzene ring. In this way, B reduces the activation energy of the reaction during the graphitization process and promotes the transformation of the amorphous carbon structure to the perfect graphite structure. Of course, this will promote crystal growth in the plane direction. The B additive promotes crystal growth, increases the degree of graphitization, and improves the graphite crystal structure. The B2O3 formed after the oxidation of the graphite sample is adsorbed on the graphite surface, and does not change the XRD peak position and peak intensity, but a separate B2O3 peak appears when the 2θ is 27.7°; the crystal apparent parameters LC and La did not change significantly. It shows that boron oxide is covered on the graphite surface as an independent phase and has no effect on the graphite structure.
The Raman spectrum of the measured graphite sample is shown in Figure 8. As the amount of H3BO3 increases, the D peak intensity gradually increases, that is, the disorder of graphite gradually increases. This is due to the transfer of B atoms to other positions after the end of graphitization. For example, the interstitial position, and the C-C bond binding force is weaker than the B-C bond, which affects the periodic arrangement of the carbon graphite structure. Therefore, an excessive amount of B atoms may cause an increase in structural disorder.
As shown in Figure 9, after oxidation treatment, as the content of H3BO3 increases, the weight loss rate of the sample gradually decreases. When the amount of H3BO3 added is higher than 3%, the graphite oxidation weight loss rate no longer decreases significantly. This is because B, as a substitute element in the graphite layer, can change the electronic structure of graphite and essentially improve the oxidation resistance of graphite. The catalytic effect of boron on graphitization is the principle of homogeneous graphitization, and B atoms uniformly replace C atoms in the graphite, so after oxidation, the active sites on the graphite surface are covered by the generated glassy B2O3, thereby preventing the further oxidation; B has a catalytic effect on graphitization, which can make more amorphous carbon become more stable graphite carbon under high temperature conditions, thereby increasing the oxidation resistance of graphite samples; when catalyzing graphitization, B replaces C, which reduces the density of active C atoms, thereby reducing O2 chemisorption; because boron atoms replace carbon atoms, the Fermi value is reduced, and the delocalized π electrons are redistributed in the direction of strengthening the C—O bond and weakening the C—C bond, thereby catalyzing the desorption of CO and CO2.
Covering the surface with B2O3 can greatly reduce the oxidation rate of carbon, so the film formed on the surface during graphite oxidation is the main reason for inhibiting oxidation. Above the melting point, B2O3 interacts with the carbon matrix and spreads along the activated carbon to form a tightly bound film. Although B2O3 does not form a continuous film, due to the strong interaction between boron and carbon atoms, a small amount of B2O3 accumulates in the active sites of carbon to inhibit it. The increase in the degree of graphitization is not the main reason for the improvement in oxidation resistance.
2.3 The influence of Ni2O3 on the oxidation resistance of graphite
Ni2O3 is produced by Shanghai Aladdin Biochemical Technology Co., Ltd., AR: 99.0%.
As shown in Figure 10, the graphite containing Ni2O3 additives is significantly different from the graphite containing Pr6O11 and H3BO3 additives after oxidation. The graphite containing Ni2O3 additive has a uniform surface after oxidation, and a lamellar structure appears. This shows that Ni is uniformly distributed in graphite and the content is small, so only the graphite phase can be observed after oxidation. This is because the graphitization temperature (2 800 ℃) is higher than the boiling point of nickel (2 732 ℃), part of the nickel volatilizes during the graphitization process, and the catalytic graphitization mechanism of nickel is the dissolution and precipitation mechanism, that is, it is first in the amorphous carbon At the interface, the C—C bond is destroyed by the strong chemical interaction between the nickel particles and the carbon material. The decomposed carbon atoms are dissolved in the nickel particles, and then precipitated out in the structure of graphite. Therefore, after graphitization is completed, the nickel is surrounded by a graphite layer, so it is difficult to detect the nickel phase.
The sample XRD data is shown in Figure 11 and Table 4. It can be seen that graphite d002 first decreases and then increases with the increase of Ni2O3 addition. When the addition of Ni2O3 is 1.0%, d002 reaches the minimum value of 0.3356 nm (very close to 0.3354 nm of natural graphite), and the highest degree of graphitization is 97.96%. Therefore, Ni obviously played a role in catalyzing graphitization.
It can be seen from Fig. 12 that compared with the blank sample, the D peak intensity of the graphite sample containing Ni2O3 additive increases, indicating that the residual Ni still affects the graphitized structure and increases the disorder of graphite. Combining with XRD data, it can be inferred that during the graphitization process, Ni uses a dissolution and re-precipitation mechanism to catalyze the graphitization of carbon, but residual Ni will destroy the graphite lattice structure and increase the degree of graphite disorder.
After oxidation treatment, the weight loss rate of the graphite sample is shown in Figure 13. The weight loss rate of the sample decreases first and then rises with the increase of the amount of Ni2O3. When the content of Ni2O3 is 3%, the weight loss rate reaches the minimum, which is 1.62%; When the Ni2O3 content is 5%, the weight loss rate of the sample is 7.43%, which has exceeded 6.05% of the blank sample. It shows that Ni can improve the oxidation resistance of graphite by catalyzing graphitization and making carbon more regular and orderly. Ni itself can promote graphite oxidation. Therefore, as the content of Ni2O3 increases, more Ni participates in the graphite oxidation reaction. And finally make the graphite weight loss rate higher than the blank sample.
3. Conclusion
1) Praseodymium oxide promotes the oxidation of graphite samples. With the increase of Pr6O11 content, the weight loss rate first increased sharply, then gradually decreased, and finally increased slowly, and its change trend was opposite to the change of graphitization degree. When the content of Pr6O11 is 0.5%, the weight loss rate of the sample is the largest, which is 17.56%; when the content is 3%, the weight loss rate of the sample is the smallest, which is 9.58%.
2) Boron has an inhibitory effect on graphite oxidation. As the amount of H3BO3 added increases, the graphite weight loss rate gradually decreases. When the amount of H3BO3 added is higher than 3%, the graphite oxidation weight loss rate no longer decreases significantly.
3) When the content of nickel oxide is small, the oxidation of graphite can be inhibited, and when the content is large, the oxidation is promoted. When the Ni2O3 content is 3%, the weight loss rate is the smallest, is 1.62%; when the Ni2O3 content is 5%, the weight loss rate of the sample is 7.43%, which has exceeded 6.05% of the blank sample contact us for more graphite news.
No related results found
0 Replies